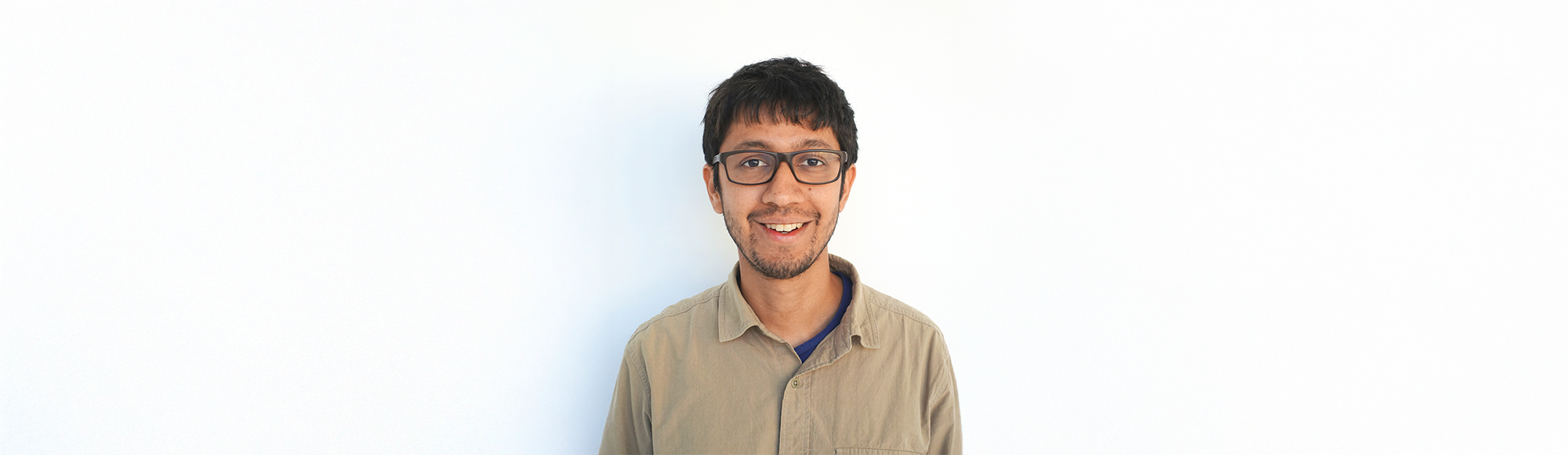
Symmetry in the Quantum World – Where Mathematics and Physics Meet
How do quantum particles organize themselves – and how can advanced mathematics help us understand nature’s deepest patterns? Apoorv Tiwari explores the invisible and the complex structures of nature itself.
As Aristotle once said, "All knowledge begins with wonder." This still holds true today, especially when we investigate the strange and fascinating world that quantum physics opens up to us. Here, nothing is quite as we know it from everyday life. Yet, it is precisely these invisible and strange mechanisms that underlie everything from the light in our lamps to the quantum computers of the future. But how do you understand something you cannot see – and that behaves completely differently from the world we know?
This is the question that Assistant Professor Apoorv Tiwari from the University of Southern Denmark seeks to answer in his research. His field of study focuses on quantum systems – systems where many tiny particles behave in ways that cannot be explained by classical physics. It is in this complex and invisible realm that he finds his professional curiosity.
"I work to understand how many quantum particles organize themselves when they are in a system together. This is important because our world – at its most fundamental nature – is quantum mechanical," explains Tiwari.
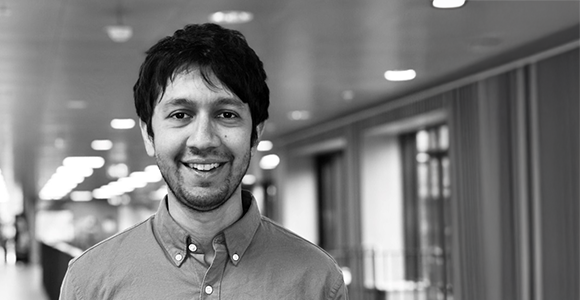
Apoorv Tiwari
Theoretical physicist and Assistant Professor at the Centre for Quantum Mathematics (QM), Department of Mathematics and Computer Science (IMADA) and the Danish Institute for Advanced Study (D-IAS). Before joining SDU, Tiwari conducted research at, among other places, the Niels Bohr International Academy at the University of Copenhagen, KTH Royal Institute of Technology in Stockholm, and as a Marie Curie Fellow at the Paul Scherrer Institute at the University of Zurich. He earned his PhD in Physics from the University of Illinois at Urbana-Champaign. His research is supported by a Villum Young Investigator grant from the Villum Foundation.
Symmetry as a Guiding Principle
But how does one work with something as complicated as quantum systems? One of the most important tools is symmetry. Most people are familiar with symmetry from geometry – something that is the same on both sides of an axis. But in physics, symmetry is about something deeper: there are patterns and rules that remain unchanged, even when we change the way we look at the system.
Symmetries have historically played a major role in the natural sciences. Newton and Kepler used them to discover laws of motion. And in the 20th century, Emmy Noether showed that symmetries are actually related to the physical laws we know – for example, that energy and electric charge are conserved.
"Symmetries don’t always provide a finished answer, but they help us eliminate what’s not possible. They make it much easier to get an overview of a system," says Tiwari.
When trying to understand how many quantum particles behave together, it is like trying to predict the movement of hundreds of billiard balls – all at once. But with quantum particles, it’s even more complex because they can be in two places at once, change suddenly, and affect each other at a distance. Here, symmetry helps bring order to chaos.
Mathematics as a Bridge to New Quantum Worlds
In recent decades, our understanding of symmetry has become more advanced. This has led to what some researchers call a symmetry revolution. One of the reasons is that today, scientists do not only look at symmetries point by point – they also look at how symmetries behave over surfaces and spaces. This is where mathematics really comes into play.
Tiwari researches how topology – a branch of mathematics that studies shapes and spaces – can be used to understand quantum systems. In topology, it is not the shape itself that is important, but how it is connected: for example, a coffee cup and a donut are considered "the same" because they both have one hole.
"My research is about finding new ways to understand symmetry and using them to investigate quantum matter. This could have major implications for, among other things, quantum computers in the future," says Tiwari.
New Phases of Matter
In school, we learn about three states of matter: solid, liquid, and gas. But in the world of quantum physics, there are many more: superconductors, superfluids, quantum magnets, and materials with so-called topological order. What they all have in common is that they don’t fit into the classical categories that physicist Lev Landau developed in the 20th century.
Since the 1980s, new quantum states have been discovered that cannot be explained by old theories. These new phases – such as Fractional Quantum Hall states, Spin Liquids, and High-Temperature Superconductors – require new ways of thinking about physics.
Tiwari is part of the new movement trying to develop an expanded classification system, using both topology and category theory (another branch of mathematics) to understand and categorize quantum states.
"My contribution is to develop the mathematical tools needed to understand these new phases. This is basic research, but it could ultimately help us build better quantum computers or develop new materials," he says.
Three new quantum states
Fractional Quantum Hall States
When electrons are squeezed into an extremely thin layer, cooled close to absolute zero, and exposed to a strong magnetic field, they start behaving as a single collective unit rather than individual particles. This creates a new kind of state where electrical charge acts as if it’s split into smaller pieces – like one-third of an electron. It’s as if nature invents entirely new kinds of particles that only exist under these extreme conditions.
Spin Liquids
In most materials, electrons point in fixed directions – like tiny compass needles. But in a spin liquid, these “needles” keep changing direction constantly, never settling. This creates a state that stays in motion even at very low temperatures. You can think of it like a magnet that never becomes fully magnetic – but where something dynamic is still happening beneath the surface.
High-Temperature Superconductors
Superconductors are materials where electricity flows without any resistance – no energy is lost as heat. Normally, this only happens at extremely low temperatures, but high-temperature superconductors can do it at slightly higher (yet still very cold) temperatures. This makes them more practical for things like powerful magnets, energy grids, or future computers. Imagine a wire where electricity flows without slowing down – that’s the idea behind superconductors.
An International Research Environment with Broad Vision
Because Tiwari’s research lies at the intersection of physics and mathematics, he is particularly happy to be part of the Centre for Quantum Mathematics.
"Here, many people are working with the exact theories and fields I am involved in – especially topological field theory and symmetries. But it’s also about collaborating with researchers from very different backgrounds. There is great interest in quantum computing, and new connections are constantly being made between theory and application. It all really fits together. This is the perfect place to be," he says.